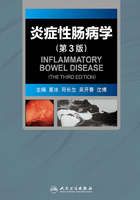
上QQ阅读APP看书,第一时间看更新
第5章 炎症性肠病的遗传学研究
第1节 炎症性肠病的遗传学研究概述
炎症性肠病(IBD)包括克罗恩病(CD)、溃疡性结肠炎(UC)以及未确定型炎症性肠病(IBD-U),是累及胃肠道的慢性炎性疾病,可导致胃肠功能减弱并可导致危及生命的并发症。尽管炎症性肠病具体的发病机制尚不清楚,但是目前认为它与遗传、环境、微生物以及免疫等因素之间复杂的相互作用相关,是遗传个体对肠道环境变化而产生失调的免疫反应的结果。
大量证据表明遗传因素在炎症性肠病的发病中有非常重要的作用。双胞胎研究发现同卵双胞胎之间的疾病一致率(CD:20%~58%,UC:14%~19%)显著高于异卵双胞胎(CD:0~7%,UC:0~7%) [1-3]。家族研究也发现炎症性肠病患病个体的一级亲属发病率为克罗恩病5%、溃疡性结肠炎1.6%。当父母双方都患病时,其后代的发病风险显著增高至大于30% [4-6]。还有,相较普通人群而言,患病个体的兄弟姐妹的风险率,克罗恩病为35%,溃疡性结肠炎为15%,这表明克罗恩病患者的家族患病风险要远远大于溃疡性结肠炎患者 [7]。
然而,比较有意思的是,炎症性肠病家族聚集现象的不同研究报道的发病一致率和相对危险度相差较大,但这表明遗传和环境因素可能共同参与了炎症性肠病的发病过程。如上所述,炎症性肠病有着较为复杂的遗传背景,即单个基因的遗传效应都比较微小,但多个易感基因与环境因素一起参与了疾病的发生和发展。
为了理解炎症性肠病多基因及多因素的本质,数项针对克罗恩病和溃疡性结肠炎的全基因组关联研究(GWAS)已经开展 [8,9],以更好地理解炎症性肠病的遗传背景。这些研究大大扩展了炎症性肠病的遗传易感基因区域,确定了超过163个的炎症性肠病的易感基因位点,而且还提示了微生物组学在炎症性肠病发病机制中的作用 [10]。通常认为这些确定的易感位点最多只能解释25%左右的预估的遗传可能性。尽管克罗恩病和溃疡性结肠炎有着不同的临床表型,但它们共享了大约30%的炎症性肠病的易感基因。
21世纪以来,全基因组关联研究建立了“共同疾病-共同突变体”模型,这也是人类基因组学的主要焦点。但是在IBD研究中,它也有其自身限制性。多疾病比较分析表明一半以上的炎症性肠病易感基因同样与其他炎性疾病或者免疫介导疾病相关,而且常常在不同的疾病中有相反的效应。例如,PTPN22 基因的编码突变R620W是类风湿关节炎和1型糖尿病的强致病因素,但它却又是克罗恩病发展的保护性因素 [11]。还有其他一些易感基因是不同但却相关疾病的共同危险因素。例如,溃疡性结肠炎与原发性硬化性胆管炎共享了数个易感基因如 MST1、IL2、CARD9和REL,而原发性硬化性胆管炎是溃疡性结肠炎的已知并发症 [12]。类似的,克罗恩病也与麻风分枝杆菌感染有共同的易感基因位点,包括 NOD2、 C13orf31、LRRK2 [13],与宿主针对分枝杆菌感染产生的免疫反应也有共同的易感基因位点,包括 CARD9、LTA、ITLN1和 IRGM [9]。这些发现提示特定的个体可能更容易发生特定的疾病,有这些重叠遗传因素的患者要比没有的患者更容易罹患并发症。
大多数GWAS对于确定大于1%的突变都是强有力的,但是对于确定更多的小于1%的罕见突变却贡献不大。因此,迄今为止,GWAS通过确定具有小遗传效应的常见等位基因(最小等位基因频率>5%),只能解释炎症性肠病总遗传效应中的很小一部分。除了NOD2,其他具有较大遗传效应的常见等位基因在炎症性肠病中尚未被报道,其他的更大规模的GWAS研究也几乎不可能有这样的发现了。同样需要注意的是,具有更高遗传效应量的高渗透性突变也从来没有被GWAS所检测到。从另外一方面讲,外显子测序可能是检测罕见突变的更有用的工具,而全基因组可能会更好地发现一些修饰基因。无论如何,炎症性肠病的许多重要的易感位点都是由GWAS发现并确认的。
基因研究以及炎症性肠病的易感基因位点研究揭示多个途径都在肠道平衡中有重要作用,这些途径包括肠道屏障功能、固有免疫和适应性免疫调控、微生物防御、上皮修复、自噬、氧自由基产生、内质网应激以及调控细胞内平衡的代谢机制等 [14]。有初步研究已经发现了与上述功能相关的致病等位基因和保护性等位基因 [15]。
第2节 肠道上皮的完整性
胃肠道管腔代表着一个非常复杂的微环境,这个微环境由食物成分以及大量的微生物及其代谢产物组成。保持微环境稳定对于维持正常的黏膜生理功能是必须的,而打破这种平衡将会引发诸多胃肠道疾病,例如炎症性肠病。肠道上皮细胞不仅仅需要发挥其营养物质吸收的基本功能,还需要利用其对管腔内容的感知能力执行屏障及信号通路功能,并且在随后还需要对其下层的固有层内容物产生适度的反应。
已经发现有一些基因具有维持屏障完整性的作用,包括 CDH1、GNA12和 PTPN2。 CDH1编码黏附结合蛋白E-黏钙素,克罗恩病患者中已经发现了这种蛋白的删减型 [16]。 GNA12编码G蛋白Gα 12,其活化导致紧密连接蛋白ZO-1和 ZO-2的磷酸化,进而导致上皮细胞系细胞之间连接失稳定 [17]。体外研究证实PTPN2能够保护细胞免受interferon-γ诱导的上皮渗透性增加,小鼠敲除模型也表明敲除该基因能够增加实验性肠炎的易感性 [18,19]。
遗传学研究已经发现了与炎症性肠病中上皮重生相关的几种转录因子,包括HNF4A和NKX2-3。HNF4A可以调控隐窝细胞增生,而NKX2-3可以调控内上皮细胞的分化 [20-22]。肠上皮细胞特定的Hnf4a敲除动物模型显示不会出现自发性肠炎,进一步为环境因素在疾病发展过程中有重要作用提供了依据 [20,21]。 STAT3基因位于IBD相关位点,其在IBD患者的上皮细胞中被激活。肠上皮特异性的 Stat3敲除被证实能够影响上皮修复 [23]。
分泌性组分对于肠道屏障完整性的重要性已经被证实。杯状细胞产生一种保护性的多糖双层,其内层是无微生物的。炎症性肠病患者有累及黏液层的倾向,并且其肠道中溶解黏液的细菌也被发现增多。小鼠模型研究中, Muc2 - / -和肠道上皮细胞特定性 C1galt1 - / -的小鼠可以观察到类似的缺陷,证明了黏液层缺陷和自发性肠炎发生之间的密切关系 [24]。一些溃疡性结肠炎患者表现出了缺陷性的o-糖基化装配,而这类似于 C1galt1 - / -小鼠的表现。潘氏细胞位于小肠隐窝,其在维持肠道干细胞环境中有重要作用。它们还分泌抗菌产物以阻断细菌侵袭,并且维持肠道微生态成分的稳定。一些与CD相关的基因如 Nod2、Atg16l1 和 Xbp1的小鼠敲除模型中可以观察到潘氏细胞缺陷及对小鼠对肠道炎症易感 [25-27]。有 ATG16L1 (T300A)突变的CD患者被发现潘氏细胞颗粒有异常。这些提示某些CD患者中潘氏细胞缺陷可能对与患者的发病有重要作用。
具有重要合成和分泌功能的细胞,如潘氏细胞和杯状细胞都有很大程度的内质网应激,引起未折叠蛋白反应(UPR)的激活,进而可以调控蛋白合成的进程。小鼠研究 [27,28]和人体研究 [27,29]都发现内质网应激增加以及未折叠蛋白反应的过度活化会有引发肠炎的倾向。这些研究提示基因突变会影响内质网应激,进而影响肠道平衡。另外,内质网应激可以激活自噬途径以及促进IL-23释放,提示增强的内质网应激可以促进由T细胞介导的炎性途径发挥作用 [29]。
第3节 遗传因素对肠道固有免疫的影响
肠道的固有免疫系统由一组高分化的细胞组成,这些细胞时刻都保护肠道免受病原微生物的侵袭,同时又可以控制避免对肠道共生菌产生高炎症反应。巨噬细胞、中性粒细胞、树突状细胞以及固有淋巴结细胞是消化道在感染和炎症状态下产生固有免疫反应的主要细胞。一些基因及其编码的蛋白被认为与肠道固有免疫密切相关,并在炎症性肠病的发病中有重要作用。
(一) NOD2和CARD9
NOD2是被发现的炎症性肠病的第一个易感基因,随后的研究又发现了与NOD2信号通路相互作用的其他基因。NOD2可以识别胞壁酰二肽(MDP),MDP既可以促进固有免疫反应,也可以促进适应性免疫反应 [30],例如可以影响自噬通路和Th17细胞分化 [31,32],且其在免疫耐受中也具有重要的作用。但这些效应在有NOD2 3020insC突变的CD患者中并没有被证实。NOD2的其他功能将在第二节中详细叙述。与炎症性肠病相关的其他固有免疫受体家族成员包括NOD-样受体(NLRs)和RIG-I样受体(RLRs),这些受体可以识别微生物或与损伤相关的分子模式,并可以激活炎性进程,因此在维持肠道平衡中有非常重要的作用。NIrP3或RIG-I敲除的小鼠极易发生实验性肠炎 [33]。
类似的, CARD9编码的适配器蛋白,可以整合来自于固有免疫受体的多个信号,识别病毒、真菌以及细菌抗原。另外, CARD9被认为同样参与了IBD的发病。在不同的条件下,CARD9与特定的信号复合体相互作用,并且适度激活不同的通路以适应不同的细胞因子微环境 [34,35]。识别真菌模式可以导致CARD9和dectin-1的产生,继而诱发广泛激活NF-κB转录家族成员的信号。而CARD9和dectin-2信号组合可以选择性地活化NF-κB因子REL,使Th17极化细胞因子IL-1β和IL-23p19亚单位表达增加 [36]。NF-κB因子REL也被认为参与了IBD的发病。
这些例子阐述了基因如 NOD2和 CARD9是如何编码固有免疫信号分子如NOD2和CARD9的。这些信号分子在整合肠道微环境的多个信号中都有至关重要的作用,并且可以选择性地活化下游效应通路,无论是在维持正常肠道健康还是在促使炎症性肠病的发展中都扮演了重要的角色。
(二) 氧化还原平衡(redox equilibrium)
肠道内的氧化还原反应参与了炎症性肠病的发病。正常的氧化还原反应依赖于氧化剂和抗氧化酶之间的平衡。氧化剂主要包括自由基、活性氧(ROS)和活性氮;抗氧化酶主要包括谷胱甘肽过氧化物酶(GPX)和谷胱甘肽-S-转移酶。小鼠模型已经证实某些特定类型GPX缺陷会导致自发性肠炎的发生。有关GPX易感基因位点的遗传学研究进一步强化了氧化还原机制在炎症性肠病发病中的作用。基础水平的ROS是无毒性的,而且是维持肠道干细胞状态的必要因素。它还在抗菌活性以及保内信号通路方面有重要作用,可以导致促炎细胞因子的产生。另外,肠道感染之后,肠上皮细胞产生的ROS可以旁分泌形式将信号传输到附近的细胞,促进并协调局部趋化因子的产生 [37]。与IBD相关的多个易感基因可以帮助调控ROS产生,保护细胞免受氧化应激。值得注意的是NOD2、CARD9以及LRRK2都可以调控ROS的产生 [30,38,39]。另外,ROS在调节性T细胞的极化和功能中也有重要的作用。因此,理解疾病的突变位点的影响将有助于进一步全面认识ROS的特定细胞和组织效应在炎症性肠病发病中的作用。
(三) 自噬
自噬功能在维持肠道平衡方面也有重要作用,主要是有:①分解及循环利用胞内细胞器和其他胞质成分;②通过其保护性的固有免疫作用来预防感染以及祛除胞内病原菌。 ATG16L1和 IRGM这两个基因被报道有自噬功能,并参与炎症性肠病的发病 [42-44]。ATG16L1在所有的自噬通路中都有着关键作用,其T300A编码突变被认为可以增加克罗恩病的发病风险。尽管 ATG16L1在多个组织中都有表达,但是截至目前此基因突变仅仅与肠道缺陷相关,这可能是因为肠道环境中有大量的微生物而机体其他大多数组织无菌。最近,更多证据表明MDP刺激诱导NOD2激活的自噬功能高度强调了易感基因之间的联系,这表明我们需要更好地理解炎症性肠病的发病机制,也需要注意发掘已知基因的未知作用 [31,32]。上皮细胞和树突状细胞中如果对与克罗恩病相关的 ATG16L1和 NOD2给予突变,会发现这些细胞的抗菌自噬功能发生了缺陷,这些缺陷可以表现为树突状细胞不能将外源性抗原递呈给CD4 +T细胞,这为上述结论提供了进一步的证据 [31,32,45]。这些发现强调了 NOD2、 ATG16L1以及自噬功能之间在调控适应性免疫系统方面的密切关系,也为这些基因能够同时影响固有免疫和适应性免疫系统提供了线索。
第4节 遗传因素对肠道适应性免疫的影响
维持促炎和抗炎成分的平衡对于维持肠道平衡至关重要,而这个过程主要依赖于适应性免疫系统各组分之间的相互作用。炎症性肠病的发生,部分原因是Th细胞发生过度的反应(克罗恩病中主要是Th1和Th17型;溃疡性结肠炎中主要是Th2型),而调节性T细胞功能下调。这种不平衡同样发生在IPEX综合征的患者中(免疫失调;多内分泌腺病;肠下垂;X-连锁病)以及Wiscott-Aldrich综合征中,同样是因为调节性T细胞功能缺陷导致肠道炎症。
调节T细胞的分化近期已被证实参与了炎症性肠病的发病。最近的数据表明Th细胞和调节性T细胞实际上起源于共同的前导体,只是在TGF-β的诱导下分化方向不一样 [46]。基于肠道有大量的TGF-β和它能通过调控T细胞发挥抗炎和促炎两个相反效应的的事实,有理由推测它是通过调控肠道固有层的幼稚CD4 +T细胞分化而发挥促平衡作用的。还有研究证实Th17细胞的促炎因子IL-6、IL17和IL23在实验性肠炎的发生中有重要作用 [47]。另外,γδ T细胞可以促进Th17相关产物的产生,导致实验性肠炎的发生,而这种肠炎可以被调节性T细胞所抑制。它们彼此之间的相互作用证明了Th17-调节性T细胞轴在胃肠道炎性环境发生和发展中的重要作用 [48,49]。
第5节 儿童炎症性肠病的遗传学研究
炎症性肠病可以在任何年龄发病,但是主要是在青春期的晚期以及青年期发病。尽管炎症性肠病的发病年龄和病程的决定因素仍未可知,但接近25%的炎症性肠病患者是在儿童期发病的 [50],而且其发病率在世界范围内还在持续上升 [51-55]。尽管证据有限,有临床证据 [56,57]表明儿童炎症性肠病患者较成人患者的临床症状更重,有更加广泛的肠道改变 [58-63]。尤其值得一提的是,儿童克罗恩病患者往往有更高的回肠和结肠瘘发病率,而儿童溃疡性结肠炎患者往往有更多的广泛结肠炎发生以及更早的第一次手术时间 [64,65]。另外,儿童克罗恩病患者中男性居多,而儿童溃疡性结肠炎患者中似乎性别差异不明显 [62]。
儿童或小儿炎症性肠病的概念是很宽泛的。目前,一般采用巴黎分类标准来有效地获得患者表型的动态特征(如病变部位和疾病表现随时间变化,生长不足等),提高儿童分类的标准,使用唯一的标准来定义炎症性肠病表型。相应的,儿童患者疾病诊断时的年龄被分为非常早期发病(VEO)或者A1a(0~10岁)以及早期发病 (EO)或A1b(10~17岁)。
但是不幸的是,至今为止的GWAS数据都没有纳入VEO炎症性肠病人群,而这个人群一般被认为有更重的病情和更大可能的阳性炎症性肠病家族史,意味着这个人群中有更高的遗传负荷和共同的遗传背景 [64,67,68]。为了扩展我们对遗传因素在炎症性肠病发病中作用的理解,研究VEO人群中低频率突变(主要是等位基因频率在0.5%~1%)情况是非常有必要的。人类基因组中的罕见基因突变不仅大大超过了常见变异 [69],而且低频率突变还被假设可以解释常见复杂疾病的大部分遗传因素 [70]。
同样,儿童炎症性肠病的发病可以是从出生到青少年晚期的任何时间。目前关于炎症性肠病早期发病是否与遗传风险增加有关仍在争论中,但是许多研究已经指出早期发病的克罗恩病患者和溃疡性结肠炎患者中家族史阳性率是增加的 [59,71]。在GWAS开展以前,关于特定基因的遗传学研究已经确定了突变与早期发生炎症性肠病之间的联系。 IBD1区域的突变便被认为与早期发生IBD相关 [72]。研究发现 IBD5基因突变与生长指数 [73]和更加严重的表型相关 [74]。同样, NOD2的一个特定突变被发现,相较成年患者而言,早期发病的克罗恩病患者有更高的基因频率。因为确定炎症开始的时间是非常困难的,因此研究儿童炎症性肠病时考虑病程因素就非常重要。儿童群体有不同的生理特征和疾病风险,以他们为研究对象比成人更有优势:①环境改变对其的影响小 [76];②由于处于活跃的生长期,年龄依赖的基因表达是唯一相关的 [77-79];③儿童有家族炎症性肠病史的可能性要大于成人;④混杂因素例如伴随疾病和药物的影响是不同的 [80-82]。因此,可以提出这样的观点,即VEO患者的病程是受更高遗传负荷 [83]以及常见遗传背景影响的 [68]。
另外,VEO炎症性肠病患者为研究初始免疫反应、早期治疗干预效果、疾病的自然病史以及早期环境改变的影响提供了机会。简而言之,儿童人群是尚未被开发但却丰富的资源,有利于获取新的以及有用的信息,以更好地理解儿童以及成人炎症性肠病的触发因素以及发病机制。为了更好地理解炎症性肠病及其风险和终极管理,更深层次的儿童基因组学是非常必要的。
自从证实了遗传因素对于炎症性肠病的贡献,大量主要针对成年人和青少年的研究就随之而开展了,因此,现有的文献广泛地描述了关于成年发生的炎症性肠病的遗传学,并将其作为主要表型 [84-87]。至今为止,所有炎症性肠病的GWAS易感位点只能解释约25%的遗传疾病风险,而且主要是白种成年人和青少年。因此,VEO人群和非欧美人群的数据是缺乏的。除了少数几个基因( NOD2、 ATG16L1、 IRGM以及 IL23R),已知的163个IBD易感基因的功能仍然不清楚。
当研究这些易感基因在早期发生的炎症性肠病中的特定影响时,许多在成年患者中确定的GWAS易感位点并不能区分早期发生与晚期发生的克罗恩病 [88]。这个结果可以导致多个结论:①已经确认的易感基因位点在早期发生和晚期发生的炎症性肠病中有相似致病性;②尽管以年龄进行了分层,病例对照研究中的表型配对干扰了早期或晚期发生的炎症性肠病的特定易感位点的确定。③早期和晚期发生的炎症性肠病中不同的表型特点是由于GWAS没有检测到的突变引起的。
许多临床病例报告和临床研究表明儿童炎症性肠病患者有不同的表型。另外,巴黎分类系统清晰地定义了VEO炎症性肠病患者中,诊断时小于10岁的儿童患者属于不同的表型(A1a) [66]。这表明采用VEO病例发现潜在的有可能解释更多重要的炎症性肠病遗传可能性。
随着二代测序(NGS)技术的快速发展和费用的降低,全外显子测序(WES)在发现功能性突变方面将会比基因面板和基因测序更加有效。另外,WES是无假设以及无偏移的方法,可以有效检测整个基因组的外显子区域以发现功能性突变。第一个应用于VEO病例的WES发现了一个可以影响调节 XIAP基因功能的罕见突变,这个病例是一名有15个月难治性炎症性肠病病程的儿童 [89]。此后,WES报道了更多的与儿童以及成人炎症性肠病相关的突变和基因。尽管有一些限制性,WES仍然是目前能够发现低频率到中频率突变的最有效的方法,相较于普通GWAS报道的突变与疾病之间非常弱的联系而言,这些突变可能具有更高的效应量。
第6节 炎症性肠病遗传因素的种族差异
现有观点常常认为西方国家的炎症性肠病患病率更高,可能提示亚洲人的炎症性肠病易感位点更少。但新的流行病学数据显示亚洲人群的炎症性肠病发病率和患病率都在稳步增长 [90,91]。既然亚洲人群的遗传因素在过去的数十年间没有发生显著性的变化,增长的炎症性肠病发病率可能提示亚洲环境因素变化对于炎症性肠病发病的重要作用。就这个角度来说,这些发现也可能提示,事实上,在炎症性肠病的发生和发展中,亚洲人与西方人有同样的遗传可能性,发病率的增长只是因为环境因素产生了变化。期望有关本领域表观遗传学的进一步研究将有助于阐释这些环境暴露因素的重要作用。相反的,另外的报道认为亚洲人群的遗传因素对炎症性肠病的影响要小于西方人群,因为在欧洲的亚洲移民中,炎症性肠病的家族聚集现象相对来说是缺少的 [92]。现阶段,关于世界范围内炎症性肠病遗传因素的差异还有许多问题有待解释清楚。
大量证据提示,不同人种和种族之间炎症性肠病易感基因位点和多态性频率确实存在不同 [93],这些突变可能是由于历史自然选择的结果。一些研究已经提示了亚洲和西方人群的炎症性肠病易感基因重要的不同之处 [94]。
西方国家的研究认为在炎症性肠病易感基因中, NOD2的常见变异Arg702Trp、Gly908Arg以及Leu1007fsinsC [95]是克罗恩病的最大危险因素 [96]。但远东亚洲人群包括日本和韩国并没有发现这些 NOD2常见突变与炎症性肠病发病相关 [95,97,98]。这个发现进一步被最近的一篇meta分析所确诊 [94]。这篇meta分析展示了日本、中国汉族、印度以及马来西亚的克罗恩病患者中主要的 NOD2易感突变是缺失的。另外,一项针对中国麻风病患者的GWAS研究确实发现 NOD2突变与麻风病相关 [13]。
类似的,西方国家研究所发现的炎症性肠病患者中自噬基因的常见突变,没有在亚洲的研究中得到证实。 ATG16L1被认为与西方国家的克罗恩病相关,日本、韩国和中国的研究了12个 ATG16L1SNPs,但没有在患者中发现这些突变 [94,99-102]。另外一个日本的研究表明 IRGM基因与炎症性肠病无关 [103],但韩国的一个独立研究又发现其与炎症性肠病相关 [104],这些矛盾但却有趣的研究使我们意识到种族间基因突变与炎症性肠病易感性之间的复杂关系,即便是在东亚遗传背景相似的亚洲人群中,这种差异也是存在的。
或许,东西方之间的炎症性肠病易感基因中差异最显著的就是 TNFSF15基因。一项日本的GWAS研究确定了这个基因,其位于染色体9q32,有5个 TNFSF15的SNPs在亚洲和欧洲患者中都被发现。但是,预期的OR值在欧洲克罗恩病患者中是1.31,在日本和韩国的患者中合并OR值高达2.39~2.84 [94,105]。
IL23/Th17通路在炎症性肠病发病中的作用同样引起了研究者的广泛关注,西方的研究提示调控这个通路的 IL23R基因与炎症性肠病的发生有密切的关系 [106]。类似的,Janus Kinase 2 ( JAK2)基因的突变已被证实与克罗恩病的发病相关,而Signal transducer and activator of transcription 3 ( STAT3)基因则被证实与克罗恩病和溃疡性结肠炎都相关 [107]。然而,东方人群 IL23R基因多态性的报道存在争议。一项韩国的研究表明2个已知的 IL23R基因突变与增高的克罗恩病发病风险相关 [100],而来自于中国和日本的研究却没有发现这样的联系 [99,108]。
炎症性肠病病理生理和遗传因素影响的另外一个重要方面就是药物基因组学,尤其是关于巯基嘌呤代谢。咪唑硫嘌呤和6-巯基嘌呤长久以来被用作治疗炎症性肠病。人们对嘌呤甲基转移酶( TPMT)基因多态性与TPMT活性下降相关以及可能与白种人骨髓毒性相关已有较多了解 [119]。比较有意思的是,亚洲人 TPMT突变体频率要比西方人群低,但是其骨髓毒性频率却又比西方人群要高 [110,111]。可能的解释是嘌呤毒性有另外的替代途径,即三磷酸肌苷焦磷酸酶(ITPA)发生缺陷。日本、中国和韩国的研究发现这些国家人群的 ITPA94C>A突变频率要高于白种人 [111-114]。
结 论
21世纪以来,关于遗传因素在炎症性肠病发病机制中的作用已被广泛研究,其中有很大一部分要归功于GWAS的成功开展。GWAS已阐释了IBD发病机制中的许多共同通路。未来对于这些通路的探索将毫无疑问会帮助我们加深对于潜在发病机制的理解,并将指导未来的研究方向。新技术例如全外显子测序可能会在本领域的未来研究中扮演重要角色。我们需要更多的努力来认识基因、环境以及微生态之间的复杂关系,以及这些因素是如何联合起来影响易感个体而导致炎症性肠病的发生的。
(Subra Koganathan 著,夏冰 审译)
参考文献
1.Tysk C,Lindberg E,Järnerot G,et al. Ulcerative colitis and Crohn’s disease in an unselected population of monozygotic and dizygotic twins. A study of heritability and the influence of smoking. Gut,1988,29 (7):990-996.
2.Thompson NP,Driscoll R,Pounder RE,et al. Genetics versus environment in inflammatory bowel disease:results of a British twin study. Bmj,1996,312 (7023):95-96.
3.Orholm M,Binder V,Sørensen TI,et al. Concordance of inflammatory bowel disease among Danish twins. Results of a nationwide study,Scand J Gastroenterol,2000,35 (10):1075-1081.
4.Orholm M,Munkholm P,Langholz E,et al. Familial occurrence of inflammatory bowel disease. N Engl J Med,1991,324:84-88.
5.Peeters M,Nevens H,Baert F,et al. Familial aggregation in Crohn’s disease:increased age-adjusted risk and concordance in clinical characteristics. Ygast,1996,111:597-603.
6.Halme L,Paavola-Sakki P,Turunen U,et al. Family and twin studies in inflammatory bowel disease. World J Gastroenterol,2006,12:3668-3672.
7.Satsangi J,Jewell DP,Bell JI. The genetics of inflammatory bowel disease. Gut,1997,40:572-574.
8.Anderson CA,Boucher G,Lees CW,et al. Meta-analysis identifies 29 additional ulcerative colitis risk loci,increasing the number of confirmed associations to 47. Nat Genet,2011,43:246-252.
9.Franke A,McGovern DP,Barrett JC,et al. Genome-wide meta-analysis increases to 71 the number of confirmed Crohn’s disease susceptibility loci. Nat Genet,2010,42:1118-1125.
10.Jostins L,Ripke S,Weersma RK,et al. Host-microbe interactions have shaped the genetic architecture of inflammatory bowel disease. Nature,2012,491:119-124.
11.Wang K,Baldassano R,Zhang H,et al. Comparative genetic analysis of inflammatory bowel disease and type 1 diabetes implicates multiple loci with opposite effects. Hum Mol Genet,2010,19:2059-2067.
12.Janse M,Lamberts LE,Franke L,et al. Three ulcerative colitis susceptibility loci are associated with primary sclerosing cholangitis and indicate a role for IL2,REL,and CARD9. Hepatology,2011,53:1977-1985.
13.Zhang FR,Huang W,Chen SM,et al. Genomewide association study of leprosy. N Engl J Med,2009,361:2609-2618.
14.Khor B,Gardet A,Xavier RJ. Genetics and pathogenesis of inflammatory bowel disease. Nature,2011,474:307-317.
15.Momozawa Y,Mni M,Nakamura K,et al. Resequencing of positional candidates identifies low frequency IL23R coding variants protecting against inflammatory bowel disease. Nat Genet,2011,43:43-47.
16.Muise AM,Walters TD,Glowacka WK,et al. Polymorphisms in E-cadherin (CDH1) result in a mis-localised cytoplasmic protein that is associated with Crohn’s disease. Gut,2009,58:1121-1127.
17.Sabath E,Negoro H,Beaudry S,et al. Galpha12 regulates protein interactions within the MDCK cell tight junction and inhibits tight-junction assembly. J Cell Sci,2008,121:814-824.
18.Scharl M,Paul G,Weber A,et al. Protection of epithelial barrier function by the Crohn’s disease associated gene protein tyrosine phosphatase n2. Gastroenterology,2009,137:2030-2040.e5.
19.Hassan SW,Doody KM,Hardy S,et al. Increased susceptibility to dextran sulfate sodium induced colitis in the T cell protein tyrosine phosphatase heterozygous mouse. PLoS ONE,2010,5:e8868.
20.Darsigny M,Babeu JP,Dupuis AA,et al. Loss of hepatocyte-nuclear-factor-4alpha affects colonic ion transport and causes chronic inflammation resembling inflammatory bowel disease in mice. PLoS ONE,2009,4:e7609.
21.Cattin AL,Le Beyec J,Barreau F,et al. Hepatocyte nuclear factor 4alpha,a key factor for homeostasis,cell architecture,and barrier function of the adult intestinal epithelium. Mol Cell Biol,2009,29 (23):6294-6308.
22.Pabst O,Zweigerdt R,Arnold HH. Targeted disruption of the homeobox transcription factor Nkx2-3 in mice results in postnatal lethality and abnormal development of small intestine and spleen. Development,1999,126:2215-2225.
23.Pickert G,Neufert C,Leppkes M,et al. STAT3 links IL-22 signaling in intestinal epithelial cells to mucosal wound healing. J Exp Med,2009,206:1465-1472.
24.Fu J,Wei B,Wen T,et al. Loss of intestinal core 1-derived O-glycans causes spontaneous colitis in mice. J Clin Invest,2011,121:1657-1666.
25.Cadwell K,Liu JY,Brown SL,et al. A key role for autophagy and the autophagy gene Atg16l1 in mouse and human intestinal Paneth cells. Nature,2008,456:259-263.
26.Biswas A,Liu YJ,Hao L,et al. Induction and rescue of Nod2-dependent Th1-driven granulomatous inflammation of the ileum. Proc Natl Acad Sci USA,2010,107:14739-14744.
27.Kaser A,Lee AH,Franke A,et al. XBP1 links ER stress to intestinal inflammation and confers genetic risk for human inflammatory bowel disease. Cell,2008,134:743-756.
28.Brandl K,Rutschmann S,Li X,et al. Enhanced sensitivity to DSS colitis caused by a hypomorphic Mbtps1 mutation disrupting the ATF6-driven unfolded protein response. Proc Natl Acad Sci USA,2009,106:3300-3305.
29.Goodall JC,Wu C,Zhang Y,et al. Endoplasmic reticulum stress-induced transcription factor,CHOP,is crucial for dendritic cell IL-23 expression,Proc. Natl. Acad. Sci. U.S. A. 107 (2010) 17698-17703.
30.Shaw MH,Kamada N,Warner N,et al. The ever-expanding function of NOD2:autophagy,viral recognition,and T cell activation. Trends Immunol,2011,32:73-79.
31.Cooney R,Baker J,Brain O,et al. NOD2 stimulation induces autophagy in dendritic cells influencing bacterial handling and antigen presentation. Nat Med,2010,16:90-97.
32.Travassos LH,Carneiro LA,Ramjeet M,et al. Nod1 and Nod2 direct autophagy by recruiting ATG16L1 to the plasma membrane at the site of bacterial entry. Nat Immunol,2010,11:55-62.
33.Zaki MH,Boyd KL,Vogel P,et al. The NLRP3 inflammasome protects against loss of epithelial integrity and mortality during experimental colitis. Immunity,2010,32:379-391.
34.Hsu YM,Zhang Y,You Y,et al. The adaptor protein CARD9 is required for innate immune responses to intracellular pathogens. Nat Immunol,2007,8:198-205.
35.Poeck H,Bscheider M,Gross O,et al. Recognition of RNA virus by RIG-I results in activation of CARD9 and inflammasome signaling for interleukin 1 beta production. Nat Immunol,2010,11:63-69.
36.Gringhuis SI,Wevers BA,Kaptein TM,et al. Selective C-Rel activation via Malt1 controls anti-fungal T(H)-17 immunity by dectin-1 and dectin-2. PLoS Pathog,2011,7:e1001259.
37.Dolowschiak T,Chassin C,Ben Mkaddem S,et al. Potentiation of epithelial innate host responses by intercellular communication. PLoS Pathog,2010,6:e1001194.
38.Gardet A,Benita Y,Li C,et al. LRRK2 is involved in the IFN-gamma response and host response to pathogens. J Immunol,2010,185:5577-5585.
39.Wu W,Hsu YM,Bi L,et al. CARD9 facilitates microbe-elicited production of reactive oxygen species by regulating the LyGDI-Rac1 complex. Nat Immunol,2009,10 (11):1208-1214.
40.Efimova O,Szankasi P,Kelley TW. Ncf1 (p47phox) is essential for direct regulatory T cell mediated suppression of CD4 + effector T cells. PLoS ONE,2011,6:e16013.
41.Kraaij MD,Savage ND,van der Kooij SW,et al. Induction of regulatory T cells by macrophages is dependent on production of reactive oxygen species,Proc. Natl Acad Sci USA,2010,107 (41):17686-17691.
42.Rioux JD,Xavier RJ,Taylor KD,et al. Genome-wide association study identifies new susceptibility loci for Crohn disease and implicates autophagy in disease pathogenesis. Nat Genet,2007,39:596-604.
43.Hampe J,Franke A,Rosenstiel P,et al. A genome-wide association scan of nonsynonymous SNPs identifies a susceptibility variant for Crohn disease in ATG16L1. Nat Genet,2007,39:207-211.
44.McCarroll SA,Huett A,Kuballa P,et al. Deletion polymorphism upstream of IRGM associated with altered IRGM expression and Crohn’s disease. Nat Genet,2008,40:1107-1112.
45.Kuballa P,Huett A,Rioux JD,et al. Impaired autophagy of an intracellular pathogen induced by a Crohn’s disease associated ATG16L1 variant. PLoS ONE,2008,3:e3391.
46.Zhou L,Lopes JE,Chong MM,et al. TGF-beta-induced Foxp3 inhibits T(H)17 cell differentiation by antagonizing RORgammat function,Nature. 2008,453 (7192):236-240.
47.Ghoreschi K,Laurence A,Yang XP,et al. Generation of pathogenic T(H)17 cells in the absence of TGF-β signalling. Nature,2010,467:967-971.
48.Do JS,Visperas A,Dong C,et al.. Cutting edge:Generation of colitogenic Th17 CD4 T cells is enhanced by IL-17+ γδ T cells. J Immunol,2011,186:4546-4550.
49.Park SG,Mathur R,Long M,et al. T regulatory cells maintain intestinal homeostasis by suppressing γδ T cells. Immunity,2010,33:791-803.
50.Loftus EV. Clinical epidemiology of inflammatory bowel disease:Incidence,prevalence,and environmental influences. Ygast,2004,126:1504-1517.
51.Kugathasan S,Judd RH,Hoffmann RG,et al. Epidemiologic and clinical characteristics of children with newly diagnosed inflammatory bowel disease in Wisconsin:a statewide population-based study. J Pediatr,2003,143:525-531.
52.Benchimol EI,Guttmann A,Griffiths AM,et al. Increasing incidence of paediatric inflammatory bowel disease in Ontario,Canada:evidence from health administrative data. Gut,2009,58:1490-1497.
53.Malaty HM,Fan X,Opekun AR,et al. Rising incidence of inflammatory bowel disease among children:a 12-year study,.J Pediatr Gastroenterol Nutr,2010,50:27-31.
54.Hope B,Shahdadpuri R,Dunne C,et al. Rapid rise in incidence of Irish paediatric inflammatory bowel disease. Archives of Disease in Childhood,2012,97:590-594.
55.Schildkraut V,Alex G,Cameron DJ,et al. Sixty-year study of incidence of childhood ulcerative colitis finds eleven-fold increase beginning in 1990s. Inflamm Bowel Dis,2013,19 (1) 1-6.
56.Hyams J,Crandall W,Kugathasan S,et al. Induction and Maintenance Infliximab Therapy for the Treatment of Moderate-to-Severe Crohn’s Disease in Children. Gastroenterology,2007,132:863-873.
57.Hyams J,Damaraju L,Blank M,et al. Induction and maintenance therapy with infliximab for children with moderate to severe ulcerative colitis. Clin Gastroenterol Hepatol,2012,10:391-9.e1.
58.Heyman MB,Kirschner BS,GOLD BD,et al. Children with early-onset inflammatory bowel disease (IBD):Analysis of a pediatric IBD consortium registry. J Pediatr,2005,146:35-40.
59.Paul T,Birnbaum A,Pal DK,et al. Distinct phenotype of early childhood inflammatory bowel disease. J Clin Gastroenterol,2006,40:583-586.
60.Gupta N,Bostrom AG,Kirschner BS,et al. Gender Differences in Presentation and Course of Disease in Pediatric Patients With Crohn Disease. Pediatrics,2007,120:e1418-e1425.
61.Van Limbergen J,Russell RK,Drummond HE,et al. Definition of Phenotypic Characteristics of Childhood-Onset Inflammatory Bowel Disease. Gastroenterology,2008,135:1114-1122.
62.Sauer CG,Kugathasan S. Pediatric inflammatory bowel disease:highlighting pediatric differences in IBD. Gastroenterol Clin North Am,2009,38:611-628.
63.Gupta N,Bostrom AG,Kirschner BS,et al. Incidence of stricturing and penetrating complications of Crohn’s disease diagnosed in pediatric patients. Inflamm Bowel Dis,2010,16:638-644.
64.Ruemmele FM,El Khoury MG,Talbotec C,et al. Characteristics of inflammatory bowel disease with onset during the first year of life. J Pediatr Gastroenterol Nutr,2006,43:603-609.
65.Thapar N,Shah N,Ramsay AD,et al. Long-term outcome of intractable ulcerating enterocolitis of infancy. J Pediatr Gastroenterol Nutr,2005,40:582-588.
66.Levine A,Griffiths A,Markowitz J,et al. Pediatric modification of the Montreal classification for inflammatory bowel disease:The Paris classification,.Inflamm Bowel Dis,2010,17:1314-1321.
67.Cannioto Z,Berti I,Martelossi S,et al. IBD and IBD mimicking enterocolitis in children younger than 2 years of age. Eur J Pediatr,2009,168:149-155.
68.Bianco AM,Zanin V,Girardelli M,et al. A common genetic background could explain early-onset Crohn’s disease. Med Hypotheses,2018,27:520-522.
69.Marth GT,Yu F,Indap AR,et al. The functional spectrum of low-frequency coding variation. Genome Biol,2011,12:R84.
70.Tennessen JA,Bigham AW,O’Connor TD,et al. Evolution and functional impact of rare coding variation from deep sequencing of human exomes. Science,2012,337:64-69.
71.Polito JM,Childs B,Mellits ED,et al. Crohn’s disease:influence of age at diagnosis on site and clinical type of disease. Ygast,1996,111:580-586.
72.Brant SR,Panhuysen CI,Bailey-Wilson JE,et al. Linkage heterogeneity for the IBD1 locus in Crohn’s disease pedigrees by disease onset and severity. Ygast,2000,119:1483-1490.
73.Russell RK,Drummond HE,Nimmo ER,et al. Analysis of the influence of OCTN1/2 variants within the IBD5 locus on disease susceptibility and growth indices in early onset inflammatory bowel disease. Gu,. 2006,55:1114-1123.
74.Brescianini S,Trinh T,Stoll M,et al. IBD5 is associated with an extensive complicated Crohn’s disease feature:implications from genotype-phenotype analysis. Gu,. 2007,56:149-150.
75.de Ridder L,Weersma RK,Dijkstra G,et al. Genetic susceptibility has a more important role in pediatric-onset Crohn“s disease than in adult-onset Crohn”s disease. Inflamm Bowel Dis,2007,13:1083-1092.
76.Cosnes J,Beaugerie L,Carbonnel F,et al. Smoking cessation and the course of Crohn’s disease:an intervention study. Ygast,2001,120:1093-1099.
77.Pott J,Stockinger S,Torow N,et al. Age-dependent TLR3 expression of the intestinal epithelium contributes to rotavirus susceptibility. PLoS Pathog,2012,8:e1002670.
78.Ramsey JM,Guest PC,Broek JA,et al. Identification of an age-dependent biomarker signature in children and adolescents with autism spectrum disorders. Mol Autism,2013,4:27.
79.Yanni SB,Smith PB,Benjamin DK,et al. Higher clearance of micafungin in neonates compared with adults:role of age-dependent micafungin serum binding. Biopharm Drug Dispos,2011,32:222-232.
80.Kappelman MD,Galanko JA,Porter CQ,et al. Association of paediatric inflammatory bowel disease with other immune-mediated diseases. Archives of Disease in Childhood,2011,96:1042-1046.
81.Román ALS,Muñoz F. Comorbidity in inflammatory bowel disease. World J. Gastroenterol,2011,17:2723-2733.
82.Pettersson B,Almer S,Albertioni F,et al. Differences between children and adults in thiopurine methyltransferase activity and metabolite formation during thiopurine therapy:possible role of concomitant methotrexate. Ther Drug Monit,2002,24:351-358.
83.Lander ES,Schork NJ. Genetic dissection of complex traits. Science,1994,265:2037-2048.
84.Lee JC,Parkes M. Genome-wide association studies and Crohn’s disease. Brief Funct Genomics,2011,10:71-76.
85.Oostenbrug LE,van Dullemen HM,te Meerman GJ,et al. IBD and genetics:new developments. Scand J Gastroenterol Suppl,2003(239):63-68.
86.Parkes M. The genetics universe of Crohn’s disease and ulcerative colitis. Dig Dis,2012,30(Suppl 1):78-81.
87.Doecke JD,Simms LA,Zhao ZZ,et al. Genetic susceptibility in IBD:overlap between ulcerative colitis and Crohn’s disease. Inflamm Bowel Dis,2013,19:240-245.
88.Essers JB,Lee JJ,Kugathasan S,et al. Established genetic risk factors do not distinguish early and later onset Crohn’s disease. Inflamm Bowel Dis,2009,15:1508-1514.
89.Worthey EA,Mayer AN,Syverson GD,et al. Making a definitive diagnosis:successful clinical application of whole exome sequencing in a child with intractable inflammatory bowel disease. Genet Med,2011,13:255-262.
90.Thia KT,Loftus EV,Sandborn WJ,et al. An update on the epidemiology of inflammatory bowel disease in Asia. Am J Gastroenterol,2008,103:3167-3182.
91.Yang SK,Yun S,Kim JH,et al. Epidemiology of inflammatory bowel disease in the Songpa-Kangdong district,Seoul,Korea,1986-2005:a KASID study. Inflamm Bowel Dis,2008,14:542-549.
92.Probert CS,Jayanthi V,Hughes AO,et al. Prevalence and family risk of ulcerative colitis and Crohn’s disease:an epidemiological study among Europeans and south Asians in Leicestershire. Gut,1993,34:1547-1551.
93.Cheon JH. Genetics of inflammatory bowel diseases:A comparison between Western and Eastern perspectives. J Gastroenterol Hepatol.,2013,28:220-226.
94.Ng SC,Tsoi KK,Kamm MA,et al. Genetics of inflammatory bowel disease in Asia:systematic review and metaanalysis. Inflamm Bowel Dis,2012,18 (6):1164-1176.
95.Croucher PJ,Mascheretti S,Hampe J,et al. Haplotype structure and association to Crohn’s disease of CARD15 mutations in two ethnically divergent populations. Eur J Hum Genet,2003,11 (1):6-16.
96.Cho JH,Brant SR. Recent insights into the genetics of inflammatory bowel disease. Gastroenterology,2011,140:1704-1712.
97.Inoue N,Tamura K,Kinouchi Y,et al. Lack of common NOD2 variants in Japanese patients with Crohn’s disease. Ygast,2002,123:86-91.
98.Lee GH,Kim CG,Kim JS,et al. Frequency analysis of NOD2 gene mutations in Korean patients with Crohn’s disease. Korean J Gastroenterol,2005,45:162-168.
99.Yamazaki K,Onouchi Y,Takazoe M,et al. Association analysis of genetic variants in IL23R,ATG16L1 and 5p13.1 loci with Crohn’s disease in Japanese patients. J Hum Genet,2007,52:575-583.
100.Yang SK,Park M,Lim J,et al. Contribution of IL23R but not ATG16L1 to Crohn’s disease susceptibility in Koreans. Inflamm Bowel Dis,2009,15:1385-1390.
101.Libioulle C,Louis E,Hansoul S,et al. Novel Crohn disease locus identified by genome-wide association maps to a gene desert on 5p13.1 and modulates expression of PTGER4. PLoS Genet,2007,3:e58.
102.Hradsky O,Dusatkova P,Lenicek M,et al. The CTLA4 variants may interact with the IL23R- and NOD2-conferred risk in development of Crohn’s disease. BMC Med Genet,2010,11:91.
103.Prescott NJ,Dominy KM,Kubo M,et al. Independent and population-specific association of risk variants at the IRGM locus with Crohn’s disease. Hum. Mol Genet,2010,19:1828-1839.
104.Moon CM,Shin DJ,Kim SW,et al. Associations between genetic variants in the IRGM gene and inflammatory bowel diseases in the Korean population. Inflamm Bowel Dis,2013,19:106-114.
105.Yang SK,Lim J,Chang HS,et al. Association of TNFSF15 with Crohn’s disease in Koreans. Am J Gastroenterol,2008,103:1437-1442.
106.Duerr RH,Taylor KD,Brant SR,et al. A genome-wide association study identifies IL23R as an inflammatory bowel disease gene. Science,2006,314:1461-1463.
107.Wang K,Zhang H,Kugathasan S,et al. Diverse genome-wide association studies associate the IL12/IL23 pathway with Crohn Disease. Am J Hum Genet,2009,84:399-405.
108.Bin C,Zhirong Z,Xiaoqin W,et al. Contribution of rs11465788 in IL23R gene to Crohn’s disease susceptibility and phenotype in Chinese population. J Genet,2009,88 (2):191-196.
109.Ansari A,Arenas M,Greenfield SM,et al. Prospective evaluation of the pharmacogenetics of azathioprine in the treatment of inflammatory bowel disease. Alimentary Pharmacology & Therapeutics,2008,28:973-983.
110.Kim JH,Cheon JH,Hong SS,et al. Influences of thiopurine methyltransferase genotype and activity on thiopurine-induced leukopenia in Korean patients with inflammatory bowel disease:a retrospective cohort study. J Clin Gastroenterol,2010,44:e242-248.
111.Kumagai K,Hiyama K,Ishioka S,et al. Allelotype frequency of the thiopurine methyltransferase (TPMT) gene in Japanese. Pharmacogenetics,2001,11:275-278.
112.Cheon JH,Kim JH,Kim BY,et al. Allele frequency of thiopurine methyltransferase and inosine triphosphate pyrophosphatase gene polymorphisms in Korean patients with inflammatory bowel diseases. Hepatogastroenterology,2009,56:421-423.
113.Cao H,Hegele RA. DNA polymorphisms in ITPA including basis of inosine triphosphatase deficiency. J Hum Genet,2002,47:620-622.
114.Marinaki AM,Sumi S,Arenas M,et al. Allele frequency of inosine triphosphate pyrophosphatase gene polymorphisms in a Japanese population. Nucleosides Nucleotides Nucleic Acids,2004,23:1399-1401.