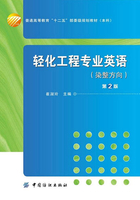
Lesson 9 Molecular Interactions of Polymers
The forces present in nature are often divided into primary forces (typically greater than 50kcal/mol of interactions) and secondary forces (typically less than 10kcal/mol of interactions). Primary bonding forces can be further subdivided into ionic (characterized by a lack of directional bonding,between atoms of largely differing electronegativities; not typically present within polymer backbones),metallic (the number of outer,valence electrons is too small to provide complete outer shells; often considered as charged atoms surrounded by a potentially fluid sea of electrons; lack of bonding direction; not typically found in polymers),and covalent (including coordinate and dative) bonding (which are the major means of bonding within polymers,directional). The bonding lengths of primary bonds are usually about 0.09-0.2nm with the carbon-carbon bond length being about 0.15-0.16nm.
Secondary forces,frequently called Van der Waals forces,since they are the forces responsible for the Van der Waals correction to the ideal gas relationships,are of longer distance in interaction generally having significant interaction between 0.25-0.5nm.① The force of these interactions is inversely proportional to some power of r,generally 2 or greater [force∝1/(distance)r] and thus is quite dependent on the distance between the interacting molecules.② Thus,many physical properties of polymers are indeed quite dependent on both the conformation (arrangements related to rotation about single bonds) and configuration (arrangements related to the actual chemical bonding about a given atom) since both affect the proximity one chain can have relative to another. Thus,amorphous polypropylene is more flexible than crystalline polypropylene [compare linear polymers (a) and (b) in Figure 9-1].
Figure 9-1 Representation of an Amorphous Polymer and Representation of Folded Polymer Chains in Polymer Crystals
Atoms in individual polymer molecules are thus joined to each other by relatively strong covalent bonds. The bond energies of the carbon-carbon bonds are on the order of 80 to 90kcal/mol. Further polymer molecules,like all other molecules,are attracted to each other (and for long-chain polymer chains even between segments of the same chain) by intermolecular,secondary forces.
These intermolecular forces are also responsible for the increase in boiling points within a homologous series such as the alkanes,for the higher than expected boiling points of polar organic molecules such as alkyl chlorides,and for the abnormally high boiling points of alcohols,amines and,amides.③ While the forces responsible for these increases in boiling points are all called van der Waals forces,these forces are subclassified in accordance with their sources and intensities. Secondary,intermolecular forces include London dispersion forces,induced permanent forces,and dipolar forces,including hydrogen bonding.
Nonpolar molecules such as ethane [H(CH2)2H] and polyethylene are attracted to each other by weak London or dispersion forces resulting from induced dipole-dipole interaction. The temporary or transient dipoles in ethane or along the polyethylene chain are due to instantaneous fluctuations in the density of the electron clouds. The energy range of these forces is about 2 kcal per mole repeat unit in nonpolar and polar polymers alike,and this force is independent of temperature. These London forces are typically the major forces present between chains in largely nonpolar polymers present in elastomers and soft plastics.
It is of interest to note that methane,ethane,and ethylene are all gases; hexane,octane,and nonane are all liquids (at room conditions); while polyethylene is a waxy solid. This trend is primarily due to both an increase in mass per molecule and to an increase in the London forces per molecule as the chain length increases.
Polar molecules such as ethyl chloride (H3C—CH2Cl) and poly(vinyl chloride)[CH2—CHCl,PVC,PAN see Figure 9-2] are attracted to each other by dipole-dipole interactions resulting from the electrostatic attraction of a chlorine atom in one molecule to a hydrogen atom in another molecule. Since this dipole-dipole interaction,which ranges from 2 to 6 kcal per mole repeat unit in the molecule,is temperature dependent,these forces are reduced as the temperature is increased in the processing of polymers. While the London forces are typically weaker than the dipole-dipole forces,they are also present in polar compounds,such as ethyl chloride and PVC. These dipole-dipole forces are characteristic of many plastics.
Figure 9-2 Typical Dipole-dipole Interaction Between Molecules of Methyl Chloride and Segments of Chains of Poly (vinyl chloride) and Polyacrylonitrile
Strong polar molecules such as ethanol,poly(vinyl alcohol),and cellulose are attracted to each other by a special type of dipole-dipole interaction called hydrogen bonding,in which the oxygen or nitrogen atoms in one molecule are attracted to the hydrogen atoms in another molecule. These are the strongest of the intermolecular forces and may have energies as high as 10kcal per mole repeat unit. Intermolecular hydrogen bonds are usually present in fibers,such as cotton,wool,silk,nylon,acrylon,polyester,and polyurethane. Intramolecular hydrogen bonds are responsible for the helices observed in starch and globular proteins.
It is important to note that the high melting point of nylon 66 (265℃) is the result of a combination of London,dipole-dipole,and hydrogen bonding forces between the polyamide chains (see Figure 9-3). The hydrogen bonds are decreased when the hydrogen atoms in the amide groups in nylon are replaced by methyl groups and when the hydroxyl groups in cellulose are esterified.
Figure 9-3 Typical Hydrogen Bonding between Hydrogen and Oxygen or Nitrogen Atoms in Nylon 66
The flexibility of amorphous polymers is reduced drastically when they are cooled below a characteristic transition temperature called the glass transition temperature (Tg). At temperature below Tg,there is no segmental motion and any dimensional changes in the polymer chain are the result of temporary distortions of the primary valence bonds. Amorphous plastics perform best below Tg,but elastomers must be used above the brittle point,or Tg.
The flexibility of amorphous polymers above the glassy state is dependent on a wriggling type of segment motion in the polymer chains. This flexibility is increased when many methylene groups (CH2) or oxygen atoms are present between stiffening groups in the chain. Thus,the flexibility of aliphatic polyesters usually increases as m is increased.
In contrast,the flexibility of amorphous polymers above the glassy state is decreased when stiffening groups such as,
are present in the polymer backbone. Thus,poly(ethylene terephthalate) is stiffer and higher melting than poly(ethylene adipate) and the former is stiffer than poly(butylene terephthalate) because of the presence of fewer methylene groups between the stiffening groups.
The melting point (Tm) is called the first-order transition temperature,and Tg is sometimes called the second-order transition temperature. The values for Tm are usually 33% to 100% greater than Tg,and symmetrical polymers like HDPE exhibit the greatest difference between Tm and Tg.
As shown in Figure 9-4,values for both Tg and Tm are observed as endothermic transitions in calorimetric measurements,such as differential thermal analysis (DTA) or differential scanning calorimetry (DSC). It is important to note that since the values observed for Tg are dependent on the test method and on time,the values obtained by different techniques may vary by a few degrees.
Figure 9-4 A Typical DTA Thermogram of a Polymer